The California sea lion has a unique way of moving through the ocean. This highly maneuverable aquatic mammal produces thrust primarily with its foreflippers – the ones it has where you have hands. Despite being fast, efficient and agile, this sea lion swimming technique is quite different from the way other large fish and marine mammals move through the water.
It wouldn’t be easy to design a system from scratch that could match the sea lion’s specifications – they produce high levels of thrust while leaving little traceable wake structure. So it makes sense to learn as much as we can about how they do it – with the thought that someday we might be able to engineer something that mimics our biological model.
To understand sea lion hydrodynamics – that is, the physics of how their swimming motion disrupts the surrounding water – we have to first characterize the kinematics – how their bodies move. And to do that we need to observe lots of California sea lion movements. So we head to the zoo.
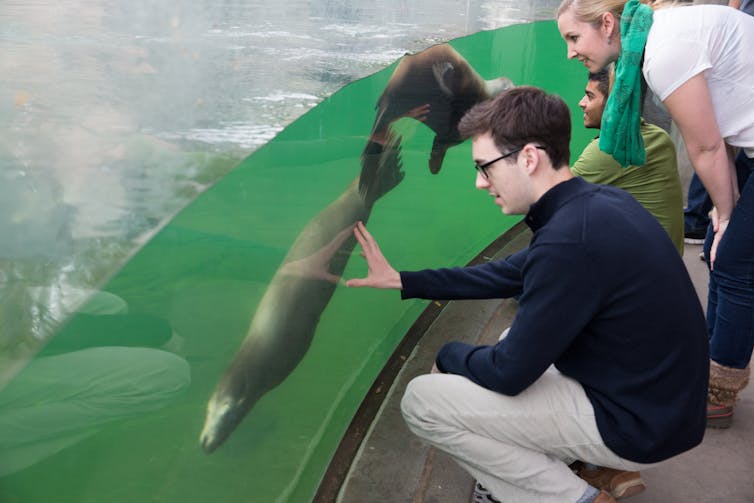
“Field” work close to home
Typically, fieldwork is hard, time-consuming and expensive. But because our “field” is only two miles away from our lab, and because the American Trail staff at the Smithsonian National Zoo is so accommodating, for us it is only hard and time-consuming.
We are able to return time and again to try new techniques and collect more data as needed. To avoid crowds but still get adequate lighting for our cameras, we arrive at 7:30 am to set up and begin taking data.
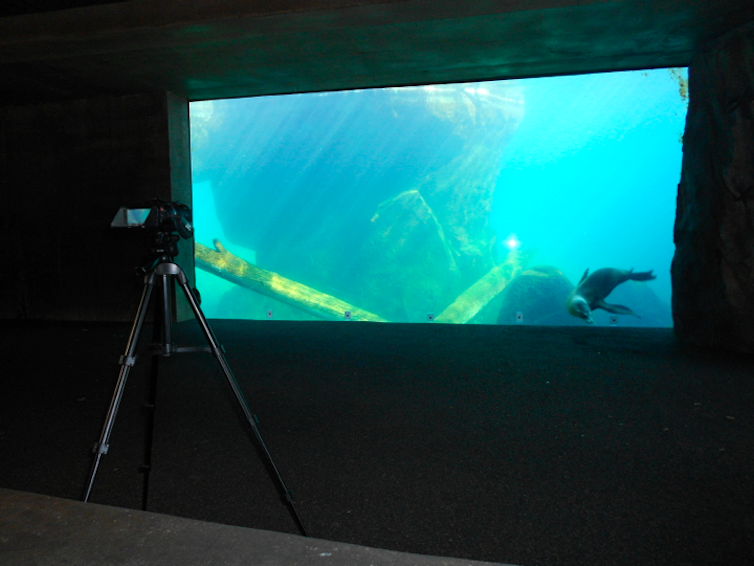
Our data are high-resolution, high-speed videos. We set up cameras in precise, known locations and place small calibration markers on the viewing window. Multiple cameras are synced using a flash or audio marker – and then we wait.
While this is an “observational” study – we do not mark or touch the animals while obtaining data – the sea lions prefer to play rather than just be observed. So we’ll wave and run back and forth across the viewing window (a great job for undergrad research interns) to entice them to exhibit the behavior we hope to capture. What we really want to see is the sea lion’s propulsive stroke – where they clap their foreflippers toward their belly and glide forward.
So far, in just under two years of collecting data, we have amassed over 100 hours of footage of sea lions swimming, about 30 minutes of which is usable data.
Back in the lab
The vast majority of our time is spent not at the zoo with the animals, but with our videos of their movements.
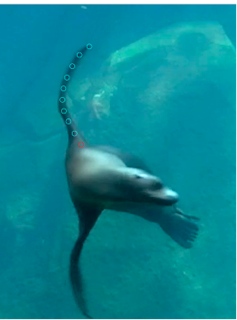
Learning something from the data we collect takes time, patience and computers. Of course video is only a two-dimensional representation of what really happened in space. So we convert all our video through a process called digital linear transformation, a method used to track three-dimensional motion that was developed by Ty Hedrick of UNC to track hummingbird and hawk moth flying.
Individual points on a sea lion’s flipper are digitally located in each frame of the video (120 frames per second). Those locations are tracked from frame to frame, creating a surface that represents the motion of the sea lion’s foreflipper while swimming.
Through this process, we can create a digital foreflipper that can be programmed to move like a real swimming California sea lion.
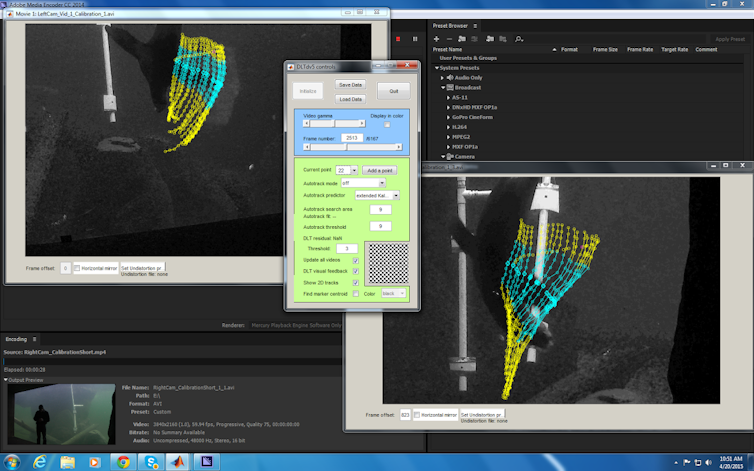
So how do they actually move?
The California sea lion relies predominantly on its foreflippers for thrust production. Thrust is the force that accelerates the animal in the forward direction. The large flippers move through the water in a clapping motion that ends with each flipper pressed against the animal’s torso.
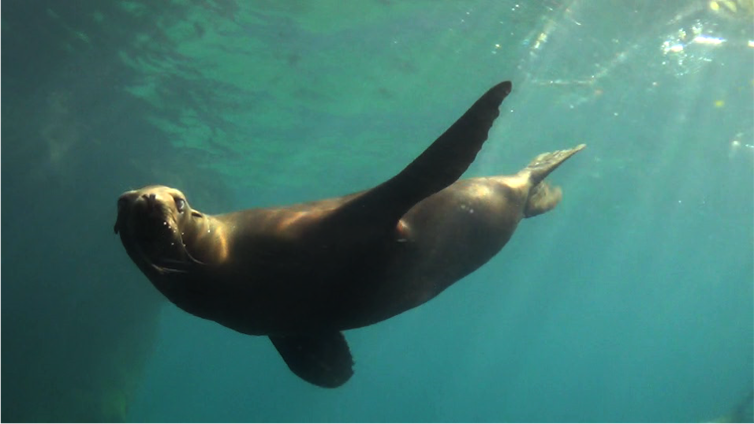
This flipper-based motion differs significantly from other large fish and marine mammals, which typically have a dominant oscillation frequency. For fish, that means they flap their tails side to side continually. Aquatic mammals flap up and down. In both, every flap takes about the same amount of time. Instead, in sea lions, each clap of the flipper is followed by a prolonged glide — particularly unusual for large, high-thrust-producing swimmers. The smooth swim is assisted by the animal’s low drag coefficient, meaning it glides through the water easily without much resistance slowing it down.
Our observational work so far has led to a detailed two-dimensional description of sea lion swimming, and we are currently working to track the flipper in three dimensions.
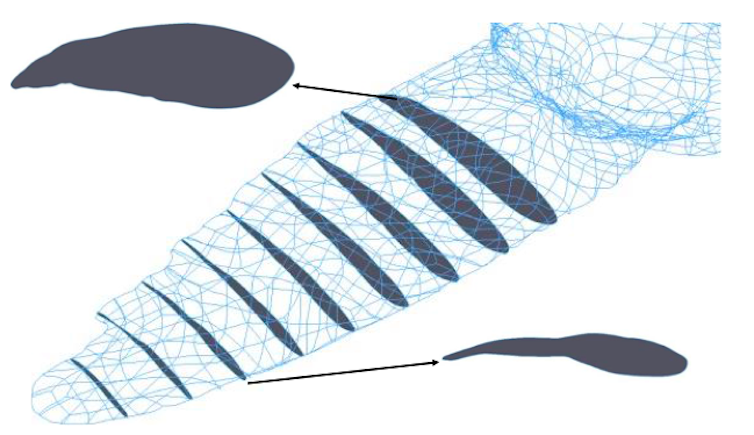
Creating a robo-foreflipper
My background, and the focus of my lab, is fluid dynamics, but so far our sea lion studies have been kinematic studies. Ultimately, we want to know how the water around the sea lion reacts to the what we’re learning about how their bodies move. To do that, we are using all the data we’ve collected from the field studies to create a robotic sea lion foreflipper.
The flipper geometry is based on high-resolution laser scans of a real California sea lion foreflipper. We program its motion based on the results of our field studies.
By creating a robotic platform, we have a controllable, scalable device that can be used in the lab. We want to measure how the water reacts to the sea lion flipper, something that’s very hard to figure out using live sea lions, mostly due to their size and the need for highly specialized equipment.
Now with our robo-foreflipper, we can investigate, and hopefully understand, the unique way that sea lions move the water while performing their one-of-a-kind swimming motion. Eventually we might see this technique incorporated into an engineered underwater vehicle that could be used to search for underwater mines, or shipwrecks, or unexplored caves – anything that requires stealth, agility and speed in the water.