The three 2018 Nobel Prize winners for chemistry were recognized for inventing fast and reliable methods for “hacking” evolution – techniques that have transformed scientific research and have already led to better drug treatments, greener and more efficient chemical manufacturing processes, and more economical biofuels.
Thanks to these inventions, what nature takes millennia to do, can now be performed by chemists in weeks or less. What’s more, these prize-winning methods form what I regard as a final proof for the molecular basis for Charles Darwin’s theory of evolution.
The Nobel Prize in chemistry was split between Frances H. Arnold and George P. Smith and Sir Gregory P. Winter; the latter two received the other half. I will admit my bias toward Frances H. Arnold whose technology the Nobel Committee recognized – on the directed evolution of enzymes, which are proteins that catalyze chemical reactions – because much of my own work has been built on it. Smith and Winter also used evolution to fast-track the development of proteins and antibodies with desirable properties. They harnessed the power of viruses to exponentially increase the scale of directed evolution envisioned by Arnold and expanded it to the development of protein-based therapeutics like Humira for chronic pain.
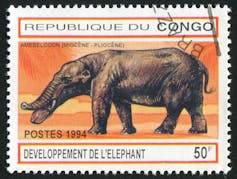
To explain it simply, both methods generate a broad variety of proteins in the lab and then use un-natural selection – that is, selecting the protein with the most desirable qualities – and then mutating this new protein in the lab to make it better and better. In that way, it’s a molecular version of evolution. Darwinian evolution created the modern elephant’s trunk from a stubby nose by the repeated processes of natural genetic mutation and survival of the fittest; directed evolution creates new enzymes from natural occurring ones by iterative cycles of mutation and selection.
Survival of the fittest - molecules
In high school biology, we learn about the “lock-and-key” concept of enzymes. In this model, enzymes, which are nature’s biocatalysts that speed up chemical reactions, are the “locks” evolved to connect with a natural target molecules – the “keys” – to complete a specific chemical reaction.
If you want an enzyme to do something new, something unnatural, like selectively insert an oxygen atom into a molecule to manufacture a valuable drug, you are unlikely to find that enzyme in nature.
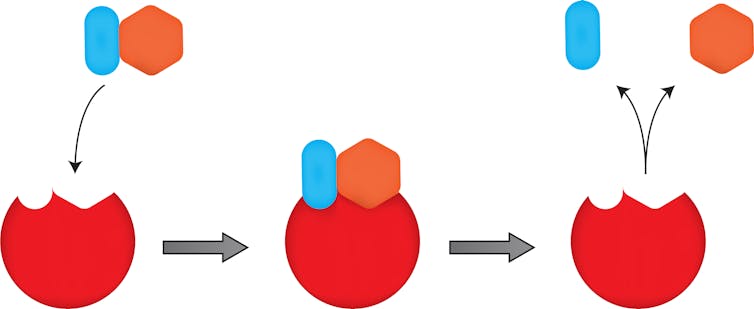
Arnold’s approach is to take an enzyme from nature and then transform it – through laboratory evolution – into one that performs the reactions that interest her. She does this by taking the gene that encodes the enzyme and putting it through the biological equivalent of an error-prone Xerox copier, which then duplicates the gene millions of times but inserts mutations randomly throughout.
Arnold then took these millions of poorly copied genes and inserted each one into a different bacterium. This collection of bacteria is called a gene library. Because each of the genes is mutated in a different way, the enzyme each bacterium produces when it is fed and grows will be slightly different. The challenge is to find the bacterium that carries the enzyme with the most desirable qualities.
For example, say you have an industrial process that requires an enzyme that works at high temperatures, but the natural enzyme falls apart under these extreme conditions. You would make thousands of random copy mutants, test them each to see if they perform at a high temperature, pick the winners and repeat the process with the winner. That’s an iterative process of mutation and selection, just like natural evolution.
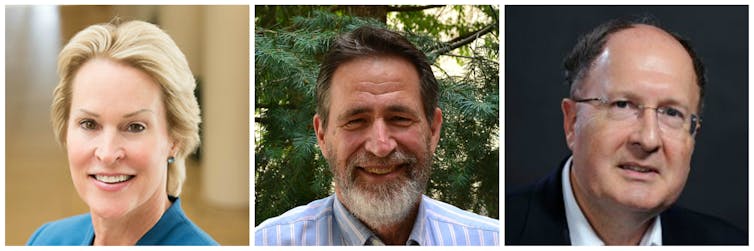
It is not unlike what it took to breed wolves into miniature dachshunds over the last tens of thousands of years. In this case, sexual reproduction is used by breeders to create gene variations and then selected traits they wanted, over many generations to arrive at the various dog breeds. Arnold figured out how to do that on a single-enzyme scale. In this way, the enzyme lock ends up changed to fit a new molecular key.
One of the first examples Arnold demonstrated as proof of concept was a little scary. She started with an enzyme responsible for drug resistance that chews up penicillin drugs and forced it to evolve to chew up a newer generation of penicillins, in this case, a more advanced antibiotic.
In this way she sped up the evolutionary clock for antibiotic resistance in a test tube. Since then, the same principle of directed evolution has been adopted to create enzymes with many useful new functions, for instance to evolve enzymes to make biofuels and drugs. Drugs now made using enzymes generated by directed evolution include the blockbuster cholesterol lowering drug atorvastatin (lipitor) and the diabetes drug sitagliptin (Januvia).
Evolution with help from viruses
Arnold shares the Nobel Prize with Smith and Winter, who invented ways to attach or “display” proteins and antibodies of interest on the surface of special virus particles, called bacteriophage. Using viruses rather than bacteria, as Arnold had done, was a different approach for identifying a gene that encoded a protein with particularly valuable qualities. This method is particularly useful for finding proteins that bind to a target protein, such as the target of a drug.
Each virus in a phage library displays a different protein on its surface. The viruses expressing the most desirable proteins are identified through several complex steps. The “winning” viruses then go through multiple cycles of mutation and testing and selection to yield proteins that fit and bind perfectly to their target.
The advantage of using viruses to display the proteins with the desired properties miniaturizes the selection process and allows you to process millions of mutated genes to find the one that best fits the job, as compared to only thousands of mutated genes using bacteria.
This technology has had the greatest impact on antibody therapies. Antibodies are molecules our immune system uses to bind and kill pathogens and naturally clear dying cells. But scientists are increasingly using them to bind to drug targets to treat various diseases. Adalimumab (Humira) is an example of a therapeutic antibody used in treatment of rheumatoid arthritis.
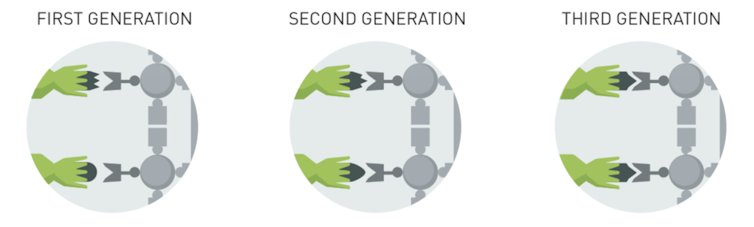
From single enzymes to evolving concerts of enzymes
I started off my own career in chemical synthesis, which is developing ways to make chemicals atom by atom in a round-bottomed flask. This is challenging work and I came to realize that organisms makes complex chemicals seemingly effortlessly as they grow, so I wanted to learn how to adapt nature’s enzyme catalysts – the tools that life uses to do chemistry – to synthesize useful unnatural molecules.
Around the mid-1990s, Arnold’s research showed me that we could ultimately use this nature-inspired method of directed evolution to improve the function of single enzymes to get them to perform chemistry they could not do naturally, millions of times faster, and get them to perform chemistry we could not do by any means.
I wondered: If doing a single biotransformation, converting A to B, is a powerful thing, could we find a way to generate pathways to connect three, four or even five or more steps, converting steps A to E and beyond in a single test tube? If one biocatalyst is a good thing, how about a molecular assembly line inside of cells for a non-natural molecule such as an AIDS drug. We called our pathway directed evolution technique bioretrosynthesis, because we start with the last step and work to the first.
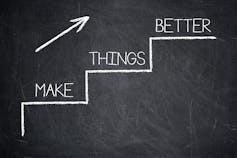
In a paper published in Nature Chemical Biology, we described how we reverse engineered a five-step molecular production line to synthesize the AIDS drug Didanosine using Arnold’s bacterial method.
Currently this drug is made using chemical processes and is very expensive. Our proof of principle showed the cost could be lessened by using a starting material that is 30-fold less expensive and using enzymes created through directed evolution to do the hard work.
One of the most exciting things to me about this Nobel breakthrough is that it provides a direct proof of Darwin’s theory of evolution, at the molecular scale, from gene to physical trait. This theory, supported by the observations of gradual changes in the fossil record over geological timescales, can now be witnessed in the lab over the course of just a few weeks to make incredibly useful tools that benefit humanity.