Quantum mechanics is a funny beast. On one hand it is a hugely successful theory, providing predictions of unrivalled accuracy across the natural world. On the other, it is a theory famed for its weirdness and failure to make sense in everyday terms: under quantum mechanics, light is both a particle and a wave, cats can be both dead and alive, and objects at the opposite ends of the galaxy exert a “spooky action-at-a-distance” on one another.
But is this weirdness really necessary? Could there be a theory more fundamental than quantum mechanics and yet in which only sensible, “non-weird” rules apply?
In particular, if we could find a theory that was “realistic”, that is, based on an intuitive reality in which objects have well-defined properties – for example dead or alive, but not both at the same time – we would solve a whole host of quantum-mechanical headaches in one swoop. But is a realistic description of nature possible? Can we have a theory which does the same job as quantum theory, and yet is free from all its weirdness?
Concrete tests
A key contribution to this discussion came from John Bell in 1960s. In addition to realism, Bell considered theories with a second very natural constraint – locality. Locality is the notion that far-apart objects cannot influence one another instantaneously. Newton described its opposite, non-locality, as “so great an Absurdity that I believe no Man who has in philosophical Matters a competent Faculty of thinking can ever fall into it”.
Bell proposed an experiment involving two particles far removed from one another. He proved that, according to all local realistic theories, a certain sequence of measurements on the two particles always return results that, when added together, give a number less than or equal to one. This relationship is known as a Bell’s inequality. Furthermore, Bell predicted that under quantum mechanics, the exact same sequence of measurements can give a value that exceeds one.
Here then was a concrete, testable difference between quantum mechanics and the whole class of theories built on local realism.
Since the initial work of John Clauser and Alain Aspect, this difference has been experimentally tested – with increasingly large distances between the particles. The result is clear: actual measurements of Bell’s ideas give a value higher than one. As this is beyond the realm of possibilities with local realistic theories, such theories cannot be viable descriptions of nature.
Doing the quantum walk
Bell’s tests involve multiple, separated objects and rule out theories which are both realistic and local. But for a single object, locality (its distance from another) isn’t an issue. So could it be that realism and realistic theories can still help us out of quantum paradoxes involving only single objects, such as Schrödinger’s cat?
This is the question addressed in new experiments by my colleagues at the University of Bonn. The team, led by Andrea Alberti, used lasers to first trap a single caesium atom and then drag it back and forth in a sequence of steps known as a “quantum walk”.
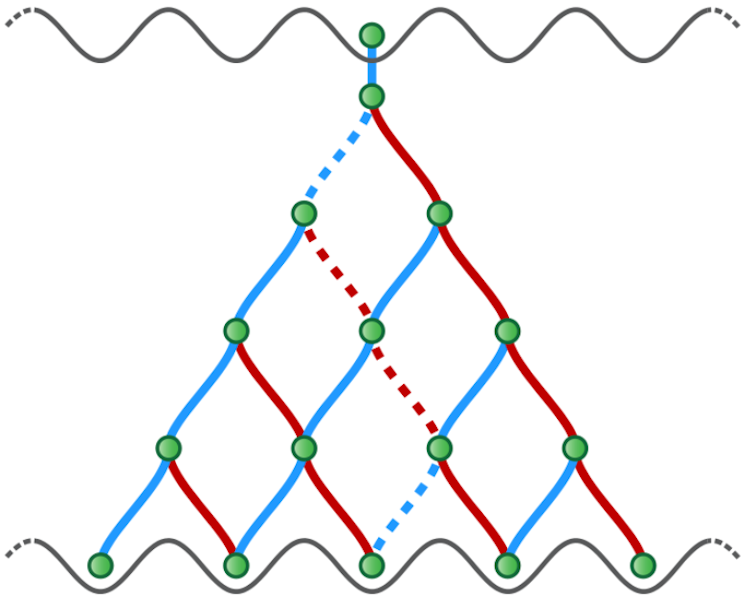
Under a realistic description of its motion, the atom has a well-defined position at every point in time, even when we are not looking at it. Provided that we also assume it’s possible to measure the atom’s position without disturbing it, this property leads to another testable inequality – the Leggett-Garg inequality.
By looking for where the atom is not, rather than where it is, the Bonn team were able to perform the most faithful Leggett-Garg test to date. Their results show a clear victory for quantum mechanics – contrary to realistic theories and our own intuition, the quantum-walking atom has to be considered as being in two places at the same time.
A quantum future
Taken together, results like these make the hope of replacing quantum mechanics with something more “sensible” appear futile. By insisting that our new theory be realistic, we inevitably end up with weird results that include the ability to act on distant objects or measurements that disrupt what they are trying to record. And these make our new theory at least as weird, if not weirder, than the quantum theory we are trying to replace.
But rather than feel disappointed at losing our easy way out from quantum’s difficulties, we should feel emboldened in our confidence in quantum mechanics. The measured violations of Bell and Leggett-Garg inequalities stem from the quantum-mechanical properties of entanglement and superposition, respectively. By embracing these properties, we are learning to construct new technologies such as quantum computing, quantum imaging and quantum encryption.
More fundamentally, future research will extend these quantum tests to include ever larger objects. We are confident that quantum mechanics holds at the smallest of scales. Likewise, a realistic description fits the behaviour of objects in our everyday experience. But somewhere in the middle, something has to give, and the sharp lines thrown by these inequalities will be central to investigating boundary between the everyday and quantum worlds.